Note: this is a repost of one of my favorite pieces from last year, when I was just getting started with this newsletter. I’m sharing to help orient new subscribers towards my general perspective on systems, and to give myself a bit of room to work on new material.
Systems Science
Systems Science is the scientific study of systems. It is about examining how systems work and seeking to understand the underlying features which regulate their dynamics.
Systems scientists consider questions such as: What is a system? What patterns of behavior exist in all types of systems? What are the features of healthy systems?
The discipline has enormous potential to improve our understanding of the complex and multifaceted issues that humanity faces. By being able to identify the patterns and casual relations that shape our world, we can begin leveraging them to our advantage.
Unfortunately, the progress of systems science as an academic discipline has been stifled. One reason for this lack of progress is the fact that systems scientists have failed to effectively communicate to those outside of the field what it is they do and how their findings can be meaningfully applied.
This piece will provide a basic overview of what systems science is. I will define it, summarize the discipline’s current state, and describe what it means to do systems science.
Defining Systems Science
Systems science is a meta-science which studies systems within all of the scientific disciplines. It aims to understand the general nature of patterns that are found in all systems.
This definition is paraphrased from George Mobus’ textbook on systems science. “Meta-science” means that it is a science of science, with insights that can be generalized to all of the scientific disciplines. Examples of patterns include hierarchy, networks, and evolution.
Physicists find these patterns in atoms, chemists find them in elements, and biologists find them in living organisms. Social scientists find them in economies and governments, while astronomers find them in the planets and stars. Systems scientists study the common features underlying all of these systems to discover what makes them tick.
Systems science is a promising candidate for the missing “dark field” that can effectively connect the rest of the traditional academic fields.

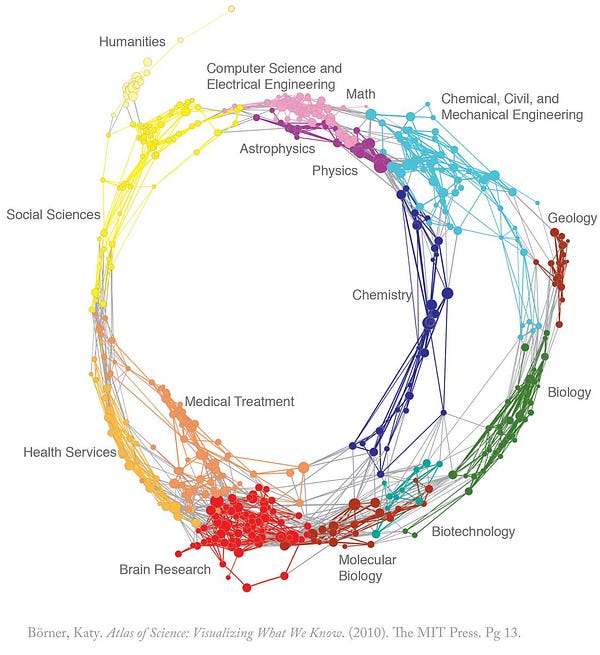
The State of Systems Science
I would describe the current state of systems science as highly disorganized and lacking unity.
There are a handful of non-profit organizations focused on systems research such as the ISSS (where I’m a member), the IFSR, and INCOSE’s working group. But there is not much effort to collaborate between the groups, they each are working towards their own ends.
There are only a small number of formal academic programs in systems science offered at universities in the world. Binghamton University, Portland State University, and the University of Ottawa are a few colleges which offer graduate programs in systems science. There is only one comprehensive textbook that has been written to accompany an introductory course in systems science. And as far as I know, this textbook isn’t actually used in any university classes.
There aren’t many people who hold the title of “Systems Scientist” within organizations. The only major exception I’ve found is Carnegie Mellon University, which employs a number of individuals who hold the title.
The result of all of this is that there aren’t many well-defined pathways for the aspiring systems scientist. These failings have prevented systems science from being accepted as a “legitimate” discipline, unlike its well-respected cousin—systems engineering.
Perhaps most importantly, there is no consensus, even within the discipline, as to what it means to do systems science.
“Systems science, as a disciplinary basis for systems understanding and systems design, has had very mixed reviews with regard to its efficacy of methods and successes in application. A central reason for this is that there is not now a single discipline of systems science that is taught as a core topic and there is not a unified concept of systems science being practiced in the field.” — Mobus, George E.. Systems Science: Theory, Analysis, Modeling, and Design (p. 98).
Doing Systems Science — Theory and Practice
Theory
Systems science is still in a phase where basic research focused on producing useful theories is desperately needed. Basic research is research that is performed without thought of practical ends. It produces general knowledge and an understanding of natural laws.
There are no broadly agreed upon, well-established principles or laws of systems. The field is missing its fundamental laws of motion or thermodynamics. Principles such as these are needed to help unify the diverse work of systems scientists around verified and widely accepted theories and terminology which apply universally to all types of systems.
Basic science is motivated by curiosity and leads to the development of general theories like Einstein’s theory of general relativity, which give us understanding but not answers to specific problems. It is a prerequisite for doing applied science which aims to answer specific questions and solve practical problems using the knowledge acquired through basic research—like detecting black holes or observing gravitational waves.
Basic research typically doesn’t lead directly to profitability while applied research often does, so there are significant economic incentives for individuals and organizations to prioritize applied science.
Until we have a better understanding of the fundamental properties of systems, systems science will have limited utility in applied science, engineering, and problem solving. Therefore, a key aspect of doing systems science today must include basic theoretical research aimed at studying many different types of systems to determine their shared properties. George Mobus, Len Troncale, Tyler Volk, and John Kinneman are a few notable systems scientists who are focused on doing this type of work.
Practice
Despite the need for work on theory, there are still plenty of opportunities for using systems science to help solve problems in practice. Computational modeling and simulation techniques such as agent-based modeling, system dynamics modeling, and network analysis are well-established as standard methods of applied systems science. These methods are especially popular in public health where they are used to study issues such as the spread of infectious disease and homelessness.
While these methods are powerful, they don’t provide a structured way of doing systems science. In order for systems science to reach its full potential it will need to develop a “systems scientific method” that establishes a shared set of procedures.
The traditional scientific method consists of the following steps:
Observe a system
Ask a question about the system
Form a hypothesis
Test the prediction with an experiment
Analyze and interpret the results
Report
One proposed framework for a “systems scientific method” is introduced by George Mobus in Systems Science: Theory, Modeling, Analysis, and Design.
System analysis
Knowledge base (created using information from analysis)
Modelling
Simulation and hypothesis testing
Generating policies and procedures
Monitoring
Process governance (to ensure this entire process is done well)
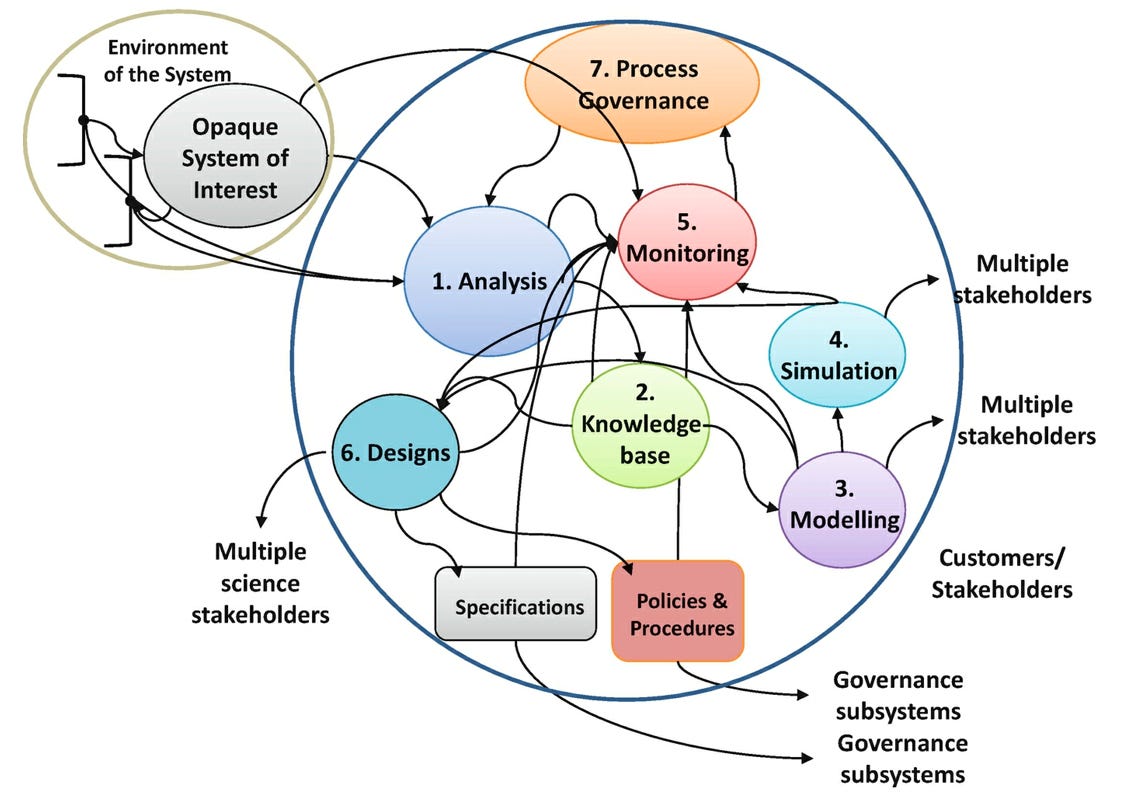
This process differs from the scientific method in that it emphasizes deep systems analysis before modeling and it assumes the incorporation of simulations. It is well-suited for gaining an understanding of complex systems where true controlled experiments are not possible. Network analysis, agent based modeling, and system dynamics modeling could be incorporated during the analysis, modeling, simulation, and monitoring stages. This method should be critiqued, improved upon, battle-tested, and met with suggestions for alternative methods that could also be employed.
One approach to doing systems science involves using the established computational methods in an unstructured manner, choosing whichever tools and procedures feel most comfortable to the researcher.
An alternative approach involves testing and developing a set of standard methods, like the one proposed by Mobus, that could be used in the same way today's scientists use the scientific method.
This second approach holds greater promise for advancing the state of systems science in the short term because it can help facilitate the establishment of widely accepted general theories for the discipline. It is easier for scientists to discover broadly agreed upon general conclusions when they are using shared procedures which allow them to repeat experiments and simulations conducted by others.
Conclusion
I’m creating Systems Explorers because I’m dismayed by the lack of societal interest in systems science. When I first read Principles of Systems Science in 2016 I knew that I wanted to study the discipline in a more formal setting with support from mentors and a community of peers. The surprising lack of resources to facilitate this exploration was discouraging. This is why I want to create this platform with the aim of bringing people together who are committed-to or intrigued-by advancing the state of systems science.
The first phase of the newsletter will be focused on theory, understanding, and fostering collaboration between people who want to advance basic research and foundational work.
Early 20th century advances in theoretical physics, driven by an intellectual desire to understand the fundamental nature of matter led to leaps in our ability to develop powerful technologies such as lasers, x-rays, nuclear power, and spaceships.
Early 21st century advances in our theoretical knowledge of systems, driven by an intellectual desire to understand their fundamental nature, will lead to leaps in our capacity to effectively coordinate. It will help us build more functional economies, stable and responsive governments, and healthier societies.
I'll start diving into the world of theoretical systems science next week by introducing the first in a series of articles looking at George Mobus’ proposed 12 principles of systems science. We’ll start by looking at his first principle of systems science—systemness.